Fusion
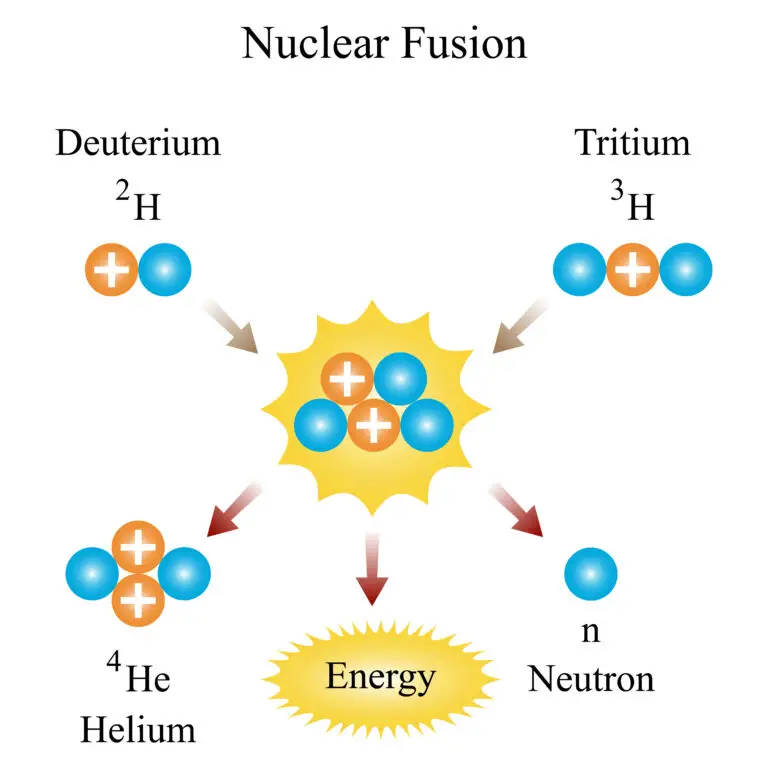
Table of Contents
What is Fusion?
Fusion is when two or more light atomic nuclei merge to create a heavier nucleus. This event releases much energy and is fundamental to powering stars, including the sun. In fusion, when the light nuclei come together, the mass of the resulting heavy nucleus is slightly less than the total mass of the original nuclei.
This “missing” mass is converted into energy, following Einstein’s famous equation E=mc^2, where E is energy, m is mass, and c is the speed of light. This equation shows that even a small amount of mass can be converted into a significant amount of energy.
Overview of Fusion
Nuclear Fusion Reaction
Light atomic nuclei merge to form a heavier nucleus in nuclear fusion, with hydrogen isotopes like deuterium (D) and tritium (T) common participants. For instance, in a typical fusion reaction, deuterium and tritium combine to create a helium nucleus, a neutron, and a significant amount of energy. The equation can summarize this process: Deuterium + Tritium → Helium + Neutron + Energy.
In this reaction, the deuterium and tritium nuclei fuse to form a stable helium nucleus (He), often helium-4. The mass of the helium nucleus and the neutron produced is less than the total mass of the fused deuterium and tritium nuclei. The “missing” mass is converted into energy, released in the reaction, and carried away by the neutron and the energy itself.
This conversion of mass to energy makes fusion a potent energy source. The neutron released in the reaction carries away excess energy. It can be utilized in further nuclear reactions, such as those in a fusion reactor, to help sustain the fusion process or generate power.
Energy Release
Fusion reactions unleash far more energy than chemical reactions due to mass conversion into energy, a process described by Einstein’s equation E=mc^2. In this equation, E stands for energy, m for mass, and c is the speed of light, which is a very large number. This relationship indicates that even a tiny mass can be converted into a huge amount of energy during fusion.
The massive energy released in fusion powers stars, including our Sun. In the cores of stars, hydrogen nuclei fuse to form helium, a process that produces the heat and light we receive from the Sun. This fusion happens under extremely high pressure and temperature conditions, where hydrogen isotopes combine to form helium and release energy through heat and radiation, sustaining the star’s luminosity and heat over billions of years.
This stellar process is a natural demonstration of the immense power of fusion, showing its potential as a nearly inexhaustible and clean energy source for Earth if it can be effectively harnessed and controlled.
Conditions for Fusion
Fusion reactions necessitate high temperatures and pressures to overcome the electrostatic repulsion between the positively charged nuclei, allowing them to get close enough for the strong nuclear force to form and bind them together. This strong nuclear force is much more powerful than the electrostatic repulsion but only operates at extremely short distances.
In the case of stars like the Sun, the immense gravitational forces generate the necessary high pressures, and the high temperatures in the core, reaching millions of degrees Celsius, provide the energy needed for hydrogen nuclei to overcome their mutual repulsion and fuse, sustaining fusion reactions.
On Earth, achieving the conditions for controlled fusion is a significant scientific and engineering challenge. Devices like tokamaks and inertial confinement fusion (ICF) systems are used in research to attain the high temperatures and densities required for fusion. Tokamaks use magnetic fields to confine and control plasma, a state of matter where electrons are stripped from atoms, creating a mix of ions and free electrons at extremely high temperatures.
Inertial confinement fusion systems, on the other hand, use lasers or ion beams to rapidly compress and heat a small target, typically containing fusion fuel, to initiate fusion. These methods aim to replicate the conditions found in stars, creating a controlled environment where fusion can occur and be studied or potentially harnessed for energy production.
Advantages and Challenges of Fusion
Advantages
- Abundant Fuel: Fusion fuels like deuterium and tritium are abundant isotopes found in water and can be extracted from seawater.
- Clean Energy: Fusion produces no greenhouse gas emissions or long-lived radioactive waste, making it a potentially clean and sustainable energy source.
- High Energy Density: Fusion reactions have a much higher energy density than chemical reactions, offering a more efficient energy source.
Challenges
- Temperature and Confinement: Achieving and maintaining the high temperatures and pressures required for fusion is technically challenging.
- Containment and Stability: Keeping the hot plasma confined and stable without losing energy to the surroundings presents engineering challenges.
- Net Energy Gain: Current fusion experiments have not yet achieved sustained net energy gain (where more energy is produced than consumed), which is essential for practical fusion power plants.
Related Links
Atomic Bomb
Neutrons
Pressure
Tension