Gravity
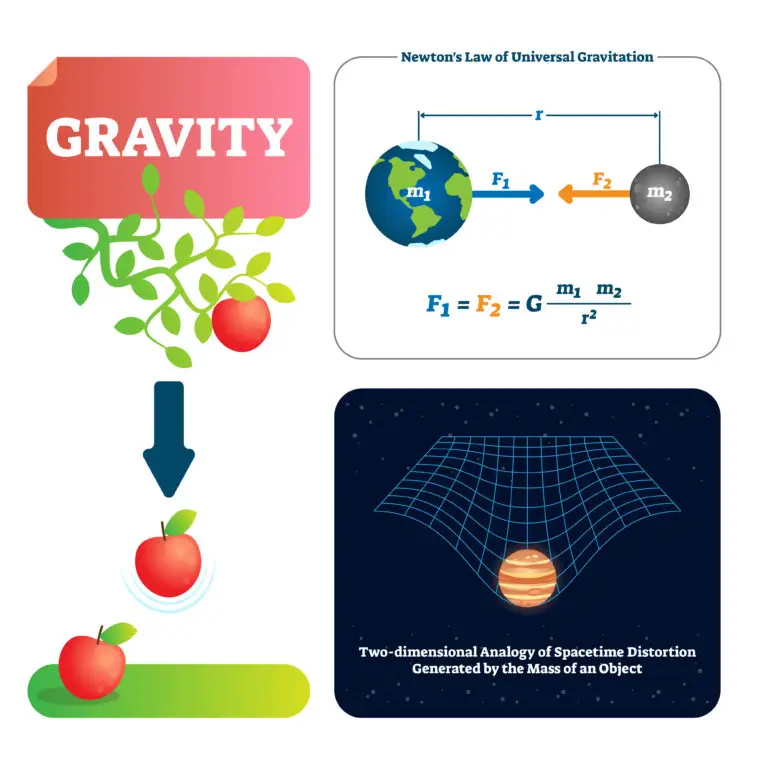
Table of Contents
What is Gravity?
Gravity is a key force in physics that causes attraction between objects that have mass. It’s one of the four fundamental forces in the universe, alongside electromagnetism, the weak nuclear force, and the strong nuclear force. Gravity is the most familiar of these forces in everyday life because it keeps us anchored to the Earth and governs the motion of planets, stars, and galaxies, holding them in their orbits.
Unlike the other three forces, typically noticeable at the atomic or subatomic levels, gravity’s effects are most evident on a large scale. It’s the weakest of the fundamental forces, yet it dominates on a cosmic level due to its long range and the fact that it only attracts, never repels. The strength of gravity between two objects depends on their masses and the distance between them: the greater the masses and the closer they are to each other, the stronger the gravitational pull.
Exploring Gravity
Nature of Gravity
Gravity is the force that pulls objects with mass toward each other. It’s a universal force acting on all objects with mass, no matter how big or small or how far apart they are. This force is the reason objects fall to the ground, planets orbit the sun, and galaxies hold together.
Isaac Newton formulated the law of universal gravitation to describe this force. According to Newton’s law, the gravitational force (F) between two objects is directly proportional to the product of their masses (m_1\text{ and }m_2) and inversely proportional to the square of the distance (r) between their centers.
This relationship is captured in the formula F=G\times \frac{m_1,m_2}{r^2}, where G is the gravitational constant, a number that quantifies the strength of the gravitational force. The law tells us that larger masses exert a stronger gravitational pull, and the force decreases rapidly as the distance between the masses increases.
Gravitational Constant (G)
The gravitational constant, denoted G, is a key quantity in physics that sets the scale of the gravitational force between objects. It is approximately 6.67430 \times 10 ^{-11} cubic meters per kilogram per second squared (m^3/kg\cdot s^2) in the International System of Units (SI). This constant is essential in the formula for calculating gravitational forces, providing the necessary link between the mass of objects and the distance between them to determine the strength of their gravitational attraction.
Effects of Gravity
- Weight: Gravity is responsible for the weight of objects on Earth. An object’s weight is the gravitational force acting on it due to Earth’s mass. Weight is measured in newtons (N) or pounds-force (lbf).
- Orbital Motion: Gravity plays a key role in the orbital motion of celestial bodies. The gravitational attraction between a planet and its moon(s) or a star and its planets causes them to orbit around each other in stable paths.
- Tides: Gravity causes tidal effects on Earth and other celestial bodies. The gravitational pull of the Moon and the Sun creates ocean tides on Earth due to the differential force exerted on different parts of the planet.
Universal Gravitation and Inverse-Square Law
Newton’s law of universal gravitation operates according to an inverse-square law, meaning that the gravitational force diminishes rapidly as the distance between two objects increases. Specifically, if you double the distance between two objects, the gravitational force between them becomes one-fourth as strong. This relationship is a key principle in gravity, indicating that while the force is powerful up close, it weakens significantly with distance.
This inverse-square nature of gravity is fundamental in the study of celestial mechanics and astrophysics. It helps scientists and astronomers understand how planets, stars, and other celestial bodies interact gravitationally.
For example, it’s crucial in calculating the orbits of planets around the sun; the farther a planet is from the sun, the weaker the sun’s gravitational pull on it, which affects how fast the planet orbits. This principle also helps determine the trajectories of spacecraft and satellites, ensuring they follow the correct paths through space.
Equivalence Principle and General Relativity
Albert Einstein’s theory of general relativity revolutionized our understanding of gravity. Instead of viewing gravity simply as a force between masses, as Newton’s laws described, Einstein proposed that massive objects cause a curvature in spacetime itself, and this curvature is what we perceive as gravity. Essentially, mass and energy tell spacetime how to curve, and the curvature of spacetime tells objects how to move.
The equivalence principle, a core concept in general relativity, posits that the effects of gravity are locally indistinguishable from the effects of acceleration. This means that being in a closed, accelerating room is indistinguishable from being in a stationary room in a gravitational field. For example, if you’re in a spaceship accelerating at 9.81 m/s² (due to Earth’s gravity), you would feel like you were standing on Earth, with everything being pulled toward the floor similarly.
This principle has deep implications for how we understand the universe. It suggests that gravity affects not just matter but also the path of light. Light passing near a massive object, like a star or a planet, will bend due to the curvature of spacetime caused by the object’s mass. This effect, known as gravitational lensing, has been observed and is one of the many confirmations of general relativity.
Related Links
Buoyancy
Density
Force
Mass