Matter
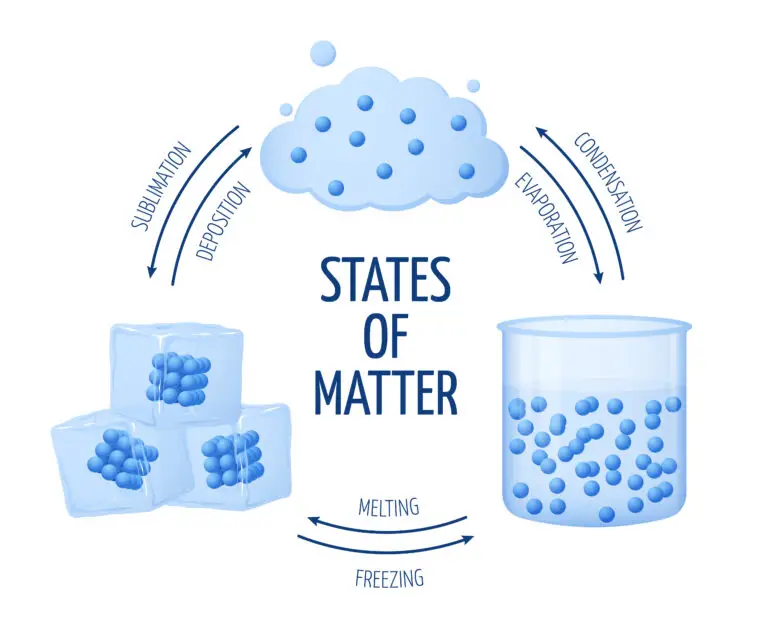
Table of Contents
What is Matter?
Matter is a substance that composes all physical objects in the universe. It’s characterized by having mass and occupying space. At the microscopic level, matter consists of various particles, including atoms, molecules, ions, and subatomic particles like protons, neutrons, and electrons. These particles form the building blocks of the different types and forms of matter we encounter.
The three traditional states of matter are solid, liquid, and gas, which differ in the arrangement and movement of their constituent particles. In solids, particles are closely packed in a fixed arrangement and vibrate in place, giving solids a definite shape and volume. Liquids have less tightly packed particles and can move around more freely, allowing liquids to take the shape of their container while maintaining a consistent volume. Gases have far apart particles that move rapidly, resulting in no fixed shape or volume, as gases expand to fill their container.
Properties of Matter
Mass
Mass indicates the amount of substance present in an object. It’s a key measure in physics and chemistry that reflects the quantity of matter contained within something, regardless of its state (solid, liquid, gas, or plasma) or position in the universe. Mass remains constant unless the matter is added to or removed from an object.
Volume
Volume is the measure of the amount of space that matter occupies. Every physical object has volume, which indicates the three-dimensional extent of the space it fills. The units used to measure volume often reflect the scale of the object or substance being measured, with cubic meters (m^3) used for larger volumes and cubic centimeters (cm^3) or milliliters (mL)—since 1cm^3 equals 1mL—used for smaller volumes.
States of Matter
Solid
In the solid state of matter, particles are tightly packed in a specific, orderly arrangement, which allows them to only vibrate around their fixed positions. This close packing and limited movement of particles give solids their distinct characteristics: a definite shape and a definite volume. Unlike liquids and gases, solids do not conform to the shape of their container; instead, they retain their own shape due to the strong intermolecular forces that hold the particles in place.
The rigidity of solids is due to these forces, which also account for other properties like hardness, brittleness, and elasticity, varying significantly among different types of solids. For example, diamonds, one of the hardest known materials, have a very strong and rigid atomic structure. In contrast, something like rubber has a more flexible molecular arrangement that allows it to stretch and deform.
Liquid
In a liquid state, particles are less tightly packed than solids and can move freely past each other. This mobility allows liquids to flow and take on the shape of their container, though they maintain a definite volume independent of the container’s shape. The intermolecular forces in liquids are strong enough to keep the particles together and maintain a consistent volume but not so strong as to fix the particles in place, as seen in solids.
This balance between cohesion (the force attracting the particles to each other) and fluidity (the ability to flow) gives liquids unique properties, such as the ability to conform to the shape of their container, a fixed volume, and a surface that is usually level.
Gas
In the gas state of matter, particles are much more spread out than solids and liquids, moving freely in all directions. This high degree of movement allows gas particles to occupy the entire volume of their container, adapting to its shape and size. Unlike solids and liquids, gases do not have a definite shape or a fixed volume; instead, they expand or compress to fill the space.
The behavior of gases is governed by various laws in physics, such as Boyle’s law, Charles’s law, and the ideal gas law, which describe the relationships between pressure, volume, temperature, and the number of particles in a gas. These properties make gases highly compressible and expansible, which is why they are used in various applications, from inflating tires to facilitating respiration in living organisms.
Plasma
Plasma is often called the fourth state of matter, distinct from the solid, liquid, and gas states. In plasma, atoms are ionized, which means their electrons are stripped away, resulting in a mixture of free-moving ions and electrons. This state occurs at very high temperatures or under strong electromagnetic fields, where the energy is sufficient to break the bonds that hold electrons to atoms.
Due to its ionized state, plasma conducts electricity and is affected by magnetic fields, making it highly dynamic and reactive. The sun is a prime example of a plasma state, where the extreme temperatures ionize hydrogen and helium, creating a hot, dense plasma that emits light and other forms of radiation. Lightning is another example of plasma created under high-energy conditions on Earth, where the rapid movement of electrons ionizes the air, leading to the familiar flash and thunder.
Classification of Matter
Pure Substances
Pure substances consist of only one type of particle and have consistent physical and chemical properties throughout. They can be categorized into elements and compounds. Elements, such as oxygen and gold, are made up of only one kind of atom and cannot be broken down into simpler substances by chemical means. Each element has unique characteristics and is represented by a symbol on the periodic table.
Conversely, compounds consist of two or more elements chemically bonded together in fixed proportions. For example, water (H₂O) is a compound of two hydrogen atoms bonded to one oxygen atom. Salt (sodium chloride, NaCl) is another compound of sodium and chlorine atoms in a 1:1 ratio. Unlike mixtures, where the component substances can vary in proportion and can often be physically separated, the components of compounds are chemically combined. They can only be separated by chemical reactions.
Mixtures
Mixtures consist of two or more substances that are physically combined without forming new chemical bonds. In mixtures, the individual substances retain their chemical identities and properties. Mixtures can be classified into two main types based on the uniformity of their composition:
Homogeneous mixtures have a consistent and uniform composition throughout. The components are mixed so thoroughly that they appear as a single phase. Solutions, like saltwater or air, are good examples of homogeneous mixtures. In these mixtures, it’s impossible to distinguish the individual components with the naked eye.
Heterogeneous mixtures contain regions or phases with different properties and compositions. These mixtures are not uniform, and the individual components can often be visually distinguished and physically separated by filtration or settling. Examples of heterogeneous mixtures include salads, sandy water, and oil and water.
Properties and Changes of Matter
Physical Properties
Physical properties are attributes of matter that can be observed or measured without altering the substance’s chemical composition. These properties do not involve a substance changing into a different substance but rather describe its physical characteristics. Examples of physical properties include:
- Color: A substance’s visual appearance or color is one of the most observable physical properties, like the yellow of gold or the red of mercury oxide.
- Density: This is a measure of mass per unit volume. It indicates how compact or concentrated matter is within a given volume. For example, lead is denser than aluminum because a given lead volume weighs more than the same volume.
- Melting point: The temperature at which a solid becomes a liquid is its melting point. This property is crucial for identifying and understanding substances’ behavior under different thermal conditions.
- Boiling point: The temperature at which a liquid turns into a gas (boils) is its boiling point. Like the melting point, the boiling point is significant for characterizing substances and is used in methods like distillation.
Chemical Properties
Chemical properties characterize the behavior of a substance when it undergoes a chemical change, resulting in the formation of new substances. These properties are intrinsic to the substance and can often only be observed or measured during a chemical reaction.
- Flammability refers to a substance’s ability to burn or ignite, causing it to react with oxygen and produce fire. For instance, gasoline is highly flammable, which is why it must be handled with care.
- Reactivity with acids is another chemical property, indicating how a substance will respond when in contact with an acid. Some metals, like zinc, react vigorously with acids, releasing hydrogen gas.
- Oxidation describes how a substance reacts with oxygen. Iron, for example, oxidizes to form rust when it combines with oxygen in water or moisture.
Energy and Matter
Matter and energy are intrinsically linked in the physical world, with their relationship governed by various processes and fundamental principles. When matter changes, such as heating and cooling, or phase changes, like melting and boiling, energy is either absorbed or released. Similarly, chemical reactions often involve energy changes, with substances absorbing energy to break bonds in reactants and releasing energy when new bonds form in products.
The law of conservation of mass and energy, one of the foundational principles of physics, states that the total amount of mass and energy in a closed system remains constant. Matter and energy can be converted into each other, but their total quantity does not change. This principle underpins the concept of mass-energy equivalence, best encapsulated in Einstein’s equation E=mc^2, where E represents energy, m is mass, and c is the speed of light. This equation shows that mass can be converted into energy and vice versa, highlighting the deep connection between the two.
Related Links
Density
Electrons
Mass
Molecule